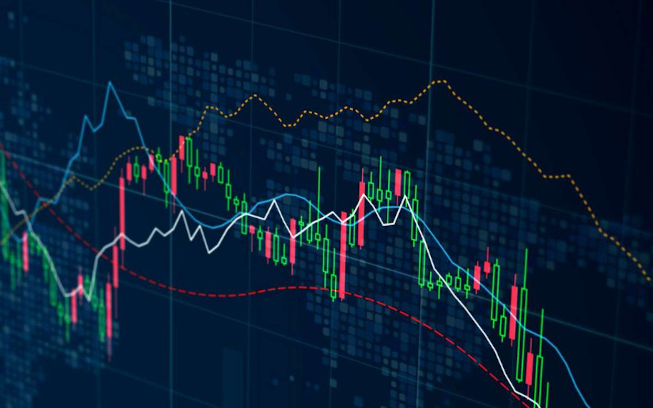
Computational Fluid Dynamics (CFD) might sound like something out of a sci-fi movie, but it’s a fascinating branch of science that plays a crucial role in many aspects of our daily lives. From designing the sleekest cars to predicting weather patterns, CFD is behind many advancements in technology and engineering. This blog post will take you on a whirlwind tour through the world of CFD, explaining what it is, cfd how it works, and why it’s so important.
The Basics of Computational Fluid Dynamics
Computational Fluid Dynamics, or CFD, is a field of study that uses numerical analysis and algorithms to solve problems involving fluid flows. In simpler terms, it’s a way to simulate how liquids and gases move and interact with their surroundings using a computer. This field is a subset of fluid mechanics, which itself is a branch of physics.
CFD is used to predict how fluid systems will behave under various conditions. For example, it can simulate how air flows around an airplane wing to improve aerodynamics or how water moves through pipes to ensure efficient transportation. By understanding fluid dynamics, engineers and scientists can design better systems and products, ensuring safety and efficiency.
The applications of CFD are vast and varied. It is widely used in industries such as automotive, aerospace, energy, environmental engineering, and more. Whether it’s designing a new car model or optimizing wind turbines, CFD plays a critical role in making informed decisions.
How CFD Works A Step-by-Step Guide
At its core, CFD relies on solving mathematical equations that describe the behavior of fluids. These equations describe various properties such as velocity, pressure, temperature, and density. However, solving these equations analytically for complex systems is incredibly difficult, if not impossible. That’s where computers come in.
The process of CFD simulation begins with defining the geometry of the system being studied. This could be anything from a simple pipe to a complex aircraft. The geometry is then divided into smaller elements, creating a mesh or grid. This step, known as discretization, breaks down the system into manageable pieces for analysis.
Once the mesh is created, the governing equations are applied to each element. These equations are solved iteratively, meaning the computer makes repeated calculations to approximate the fluid’s behavior. By adjusting variables and refining the mesh, the simulation becomes more accurate over time.
The Importance of Meshing and Discretization
Meshing is a critical step in CFD as it determines the level of detail and accuracy of the simulation. A finer mesh with more elements provides better resolution and results but requires more computational power and time. Conversely, a coarser mesh may be quicker to compute but might oversimplify the system.
The art of meshing involves finding the right balance between accuracy and computational efficiency. Engineers must consider factors like the complexity of the geometry, desired level of detail, and available computational resources. Properly meshing a model ensures that the simulation accurately captures the physics of fluid flow.
Discretization, a closely related concept, involves approximating continuous equations with discrete values. Since computers can’t handle continuous data, the equations are transformed into algebraic forms that can be solved numerically. This approach allows CFD to tackle complex problems that would be impractical to solve otherwise.
Navier-Stokes Equations The Heart of CFD
At the core of CFD simulations lie the Navier-Stokes equations. These partial differential equations describe how fluids behave and evolve over time. Named after Claude-Louis Navier and George Gabriel Stokes, these equations take into account factors like viscosity, pressure, and external forces.
The Navier-Stokes equations are notoriously challenging to solve due to their non-linear nature. For most real-world applications, analytical solutions don’t exist, which is why numerical methods like CFD are employed. By breaking down the equations into smaller pieces and using computers to perform countless calculations, engineers can gain valuable insights into fluid behavior.
While the Navier-Stokes equations are fundamental, CFD simulations often involve additional equations to account for turbulence, heat transfer, and chemical reactions. These supplementary equations add complexity but are necessary to accurately model real-world scenarios.
Applications of CFD in Aerospace Engineering
In the aerospace industry, CFD is an indispensable tool for designing aircraft and spacecraft. Engineers use CFD simulations to study how air flows around wings, fuselages, and engines. By understanding the aerodynamics, they can optimize designs for better performance, fuel efficiency, and safety.
CFD also plays a crucial role in predicting airflow within an aircraft cabin, ensuring passenger comfort and safety. Simulations can help identify areas of turbulence and improve ventilation systems. In spacecraft design, CFD is used to analyze aerodynamic heating during re-entry, a critical aspect of ensuring structural integrity.
The ability to simulate and visualize airflow patterns allows aerospace engineers to make informed decisions without relying solely on costly physical prototypes. This significantly accelerates the design process and reduces development costs.
Automotive Industry Fueling Innovation with CFD
In the world of automobiles, CFD has revolutionized how cars are designed and tested. Engineers use CFD to analyze airflow around vehicles, enabling them to create more aerodynamic shapes that improve fuel efficiency and reduce drag. This leads to vehicles that are not only faster but also more environmentally friendly.
CFD is also applied to study cooling systems, ensuring engines operate at optimal temperatures. By simulating heat transfer and fluid flow, engineers can design efficient radiators and cooling ducts, enhancing the overall performance of the vehicle.
Furthermore, CFD helps optimize automotive interiors by analyzing airflow and temperature distribution. This ensures passenger comfort while reducing the need for excessive air conditioning, ultimately improving energy efficiency.
Energy Sector Harnessing CFD for Efficiency
The energy sector relies heavily on CFD to optimize various processes and systems. In the realm of renewable energy, CFD is used to design and improve wind turbines. By simulating airflow and turbulence, engineers can maximize energy generation while minimizing structural stress.
In the oil and gas industry, CFD plays a vital role in optimizing drilling operations and pipeline transport. Simulations help engineers understand how fluids move through complex networks, allowing them to design efficient and safe systems.
CFD is also applied to combustion analysis in power plants, optimizing fuel combustion and reducing emissions. This leads to cleaner and more efficient energy production, aligning with global sustainability goals.
Environmental Engineering Protecting the Planet with CFD
Environmental engineers use CFD to address various environmental challenges, from pollution control to wastewater treatment. By simulating pollutant dispersion in the atmosphere or water bodies, engineers can develop strategies to minimize environmental impact.
CFD is instrumental in designing efficient ventilation systems for buildings, ensuring optimal indoor air quality. Simulations help engineers understand airflow patterns and identify areas of poor ventilation, allowing for targeted improvements.
In the field of hydrodynamics, CFD is used to study water flow in rivers, lakes, and oceans. This information is crucial for flood management, sediment transport, and aquatic ecosystem preservation.
Medical and Biological Applications of CFD
In the realm of medicine and biology, CFD is making significant contributions to understanding complex physiological processes. For instance, CFD is used to simulate blood flow in arteries, aiding in the diagnosis and treatment of cardiovascular diseases. By analyzing how blood moves through the vascular system, doctors can make informed decisions about interventions and surgeries.
CFD also plays a role in designing medical devices and implants. Engineers use simulations to optimize the performance of devices like stents, ensuring they work effectively within the human body. This reduces the need for costly and time-consuming physical testing.
Furthermore, CFD is applied in respiratory research to study airflow in the lungs. Simulations help researchers understand how air moves through the respiratory system, providing insights into conditions like asthma and chronic obstructive pulmonary disease (COPD).
Challenges and Limitations of CFD
While CFD offers incredible insights and capabilities, it also comes with its share of challenges. One of the primary limitations is the computational cost. Simulating complex fluid systems requires significant computing power and time, limiting the feasibility of certain simulations.
Additionally, accurately modeling turbulence is a persistent challenge in CFD. Turbulence is a chaotic and unpredictable phenomenon, making it difficult to capture in simulations. Despite advances in turbulence modeling, it remains an area of active research.
Furthermore, CFD results are only as accurate as the input data and assumptions made during simulation. Errors in geometry, boundary conditions, or material properties can lead to inaccurate predictions. Therefore, careful validation and verification are essential to ensure reliable results.
The Future of CFD Emerging Trends and Innovations
The future of CFD holds exciting possibilities as technology continues to advance. One promising trend is the integration of artificial intelligence (AI) and machine learning into CFD simulations. AI algorithms can enhance the accuracy and speed of simulations by learning from large datasets and identifying patterns.
Another area of innovation is the development of more powerful and efficient computational methods. High-performance computing (HPC) and cloud-based solutions are making CFD accessible to a broader range of industries and researchers.
Additionally, advancements in visualization techniques are enhancing the interpretation of CFD results. Interactive and immersive visualization tools allow engineers to explore complex fluid dynamics intuitively, leading to more informed decision-making.
Conclusion The Impact and Potential of CFD
In conclusion, Computational Fluid Dynamics is a remarkable field that has transformed industries and scientific research alike. From aerospace to medicine, CFD plays a pivotal role in understanding and manipulating fluid behavior, driving innovation, and solving complex problems.
By leveraging the power of computers and mathematics, engineers and scientists can simulate real-world scenarios with unprecedented accuracy. This enables better designs, improved efficiency, and enhanced safety across a wide range of applications.
As technology continues to evolve, the potential of CFD is boundless. The integration of AI and advanced computational methods will further expand its capabilities, opening new frontiers in fluid dynamics research. Whether you’re an engineer, scientist, or simply curious about the science behind fluid motion, CFD offers a fascinating glimpse into the world of fluid mechanics.
With its profound impact on modern engineering and scientific discovery, CFD remains an indispensable tool for shaping a more innovative and efficient future.